Yale-led team develops shape-shifting turtle robot
A group of researchers led by Professor of Mechanical Engineering and Materials Science Rebecca Kramer-Bottiglio have developed an amphibious robotic turtle that can adapt to disparate environments.
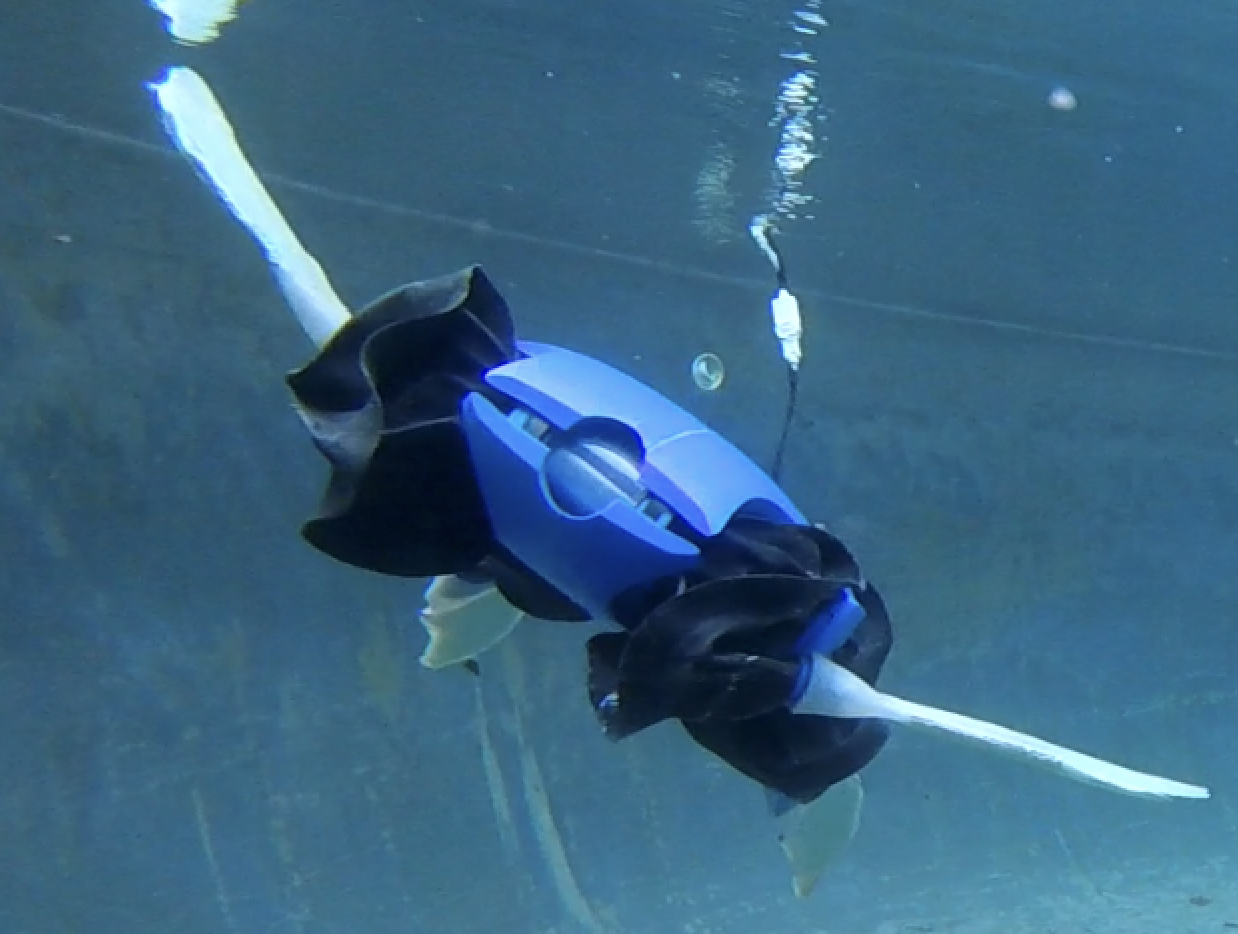
Courtesy of Robert Baines
Yale researchers have developed an amphibious robot, complete with shape-shifting limbs that can outperform both legs on land and flippers in water.
From cleaning houses, to managing warehouses, to exploring extreme terrains, the expanding diversity of mobile robotic applications has increased the types of environments that robots must be equipped to face. In their paper published in the journal Nature, a team of Yale researchers developed a robotic turtle with morphing limbs that can travel effectively through disparate natural environments. This study was guided by principal investigator Rebecca Kramer-Bottiglio, the John J. Lee Associate Professor of mechanical engineering and materials science.
“Animals can’t adapt their physiology radically to change the way they move in their environment,” Robert Baines GRD ’23, one of the paper’s co-first authors, and a doctoral researcher in mechanical engineering and materials science, told the News. “What if we could have evolution on demand?”
Most animals and robots alike are specialized to specific environments — their locomotion is designed to be efficient in a particular niche, which comes at the expense of performance in others. For instance, swimming with giraffe legs would prove quite a challenge, while walking with dolphin flippers sounds equally improbable.
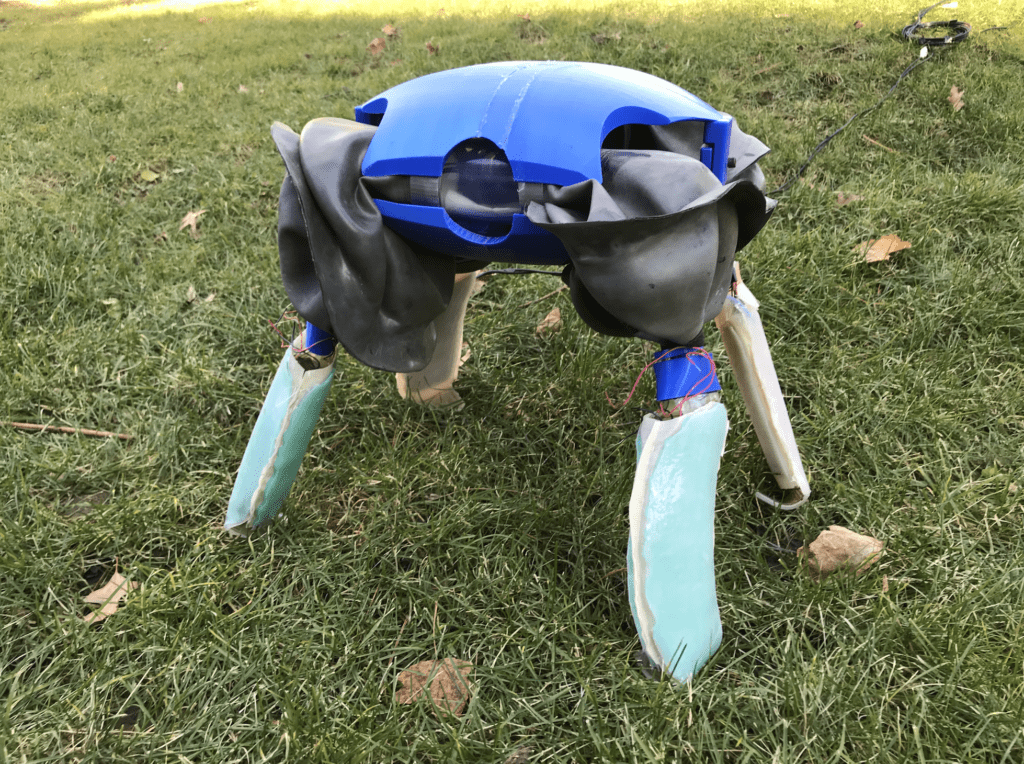
To design a robot that can thrive in both worlds, the researchers studied the natural movement mechanisms of terrestrial and aquatic turtles in their respective elements, then integrated them through a design philosophy dubbed “adaptive morphogenesis.”
Morphogenesis alone refers to the emergence of form, or how an animal takes its shape. In natural organisms, this process and the resulting shapes are largely fixed, at least in the window of individual lifetimes. Baines explained that adaptive morphogenesis can be thought of as evolution on demand — changing form in a much shorter timeframe to adapt to the surrounding environment.
Considering the fixed anatomies of animals, using adaptive morphogenesis to change limb shapes and movement patterns would only be possible for an artificial robotic system.
“The main takeaway of the paper isn’t the specific robot embodiment, but rather the design philosophy,” Baines said.
From this design philosophy emerged the Yale researchers’ amphibious robotic turtle (ART), whose morphing limbs are capable of transforming between legs and flippers. Sree Patiballa — the paper’s other co-first author and a former Yale postdoctoral researcher — described the legs as semi-circular in shape with a blunt end, advantageous for bearing the robot turtle’s weight on land. In flipper mode, the limbs become longer and flatter, ideal for propulsion in water.
Designing and constructing ART’s shape-shifting limbs was no easy feat. While many parts like the shell and joints are 3D-printed, the limbs are fabricated with materials that can radically change stiffness by a factor of 450 times.
This variable stiffness allows for a change in limb shape when needed, but remains otherwise rigid and holds the desired shape. Without the ability to change their stiffness, the limbs would either be too rigid to morph, or too flimsy for efficient propulsion in water and weight-bearing on land.
“The variable-stiffness material is thermally responsive and holds the [limb] shape, so we don’t have to keep on pumping the pressure,” Patiballa said. “Once it cools down, we deflate the pressure, and the limb still retains that shape. Once you reheat it, it softens and goes back to the flipper shape.”
In addition to changing limb shapes, ART can also change its movement patterns, or “gaits,” depending on the environment, since the mechanisms of motion are different in water compared to on land. For instance, trying to walk while submerged or paddle on land would prove very difficult. The researchers created the robot for three distinct environments: land, water and the transition zone in between.
Using twelve motors in ART’s shoulder joints, three per limb, the researchers programmed four gaits specialized to these conditions: walking, crawling, flapping and paddling.
Baines described the robot’s walking as the “creep” gait, where ART keeps three legs on the ground at all times to remain statically stable. ART’s walking was tested over diverse terrestrial terrains, from predictable lab settings to outdoor environments such as a paved sidewalk or sandy, pebbly turf. The crawling gait, similar to a beaching sea turtle, is designed for the transition zones between land and water, where ART lies on its stomach and pulls itself forward.
Swimming was divided into two separate gaits — flapping and paddling, modeled after sea and freshwater turtles, respectively. Flapping is more efficient for propulsion when ART is fully submerged, while paddling near the surface yields better acceleration and heightened maneuverability. Changing the angle of the flippers, along with the robot’s buoyancy, can make the robot change its depth by diving or surfacing.
Ever since the first prototype was built in 2019, the researchers, each with their own unique skill sets, have been enhancing and optimizing the robot. Waterproofing the system to prevent damage to the motors, electronics and other internal components was one of the difficult challenges they faced early on. Patiballa described the successful control of ART’s buoyancy as another major milestone. The slightest modification in buoyancy could result in ART quickly sinking to the bottom of a pool or floating up to the top — finding a balance proved to be crucial for submerged swimming.
“Mechanical designers, experts in materials science and biologists who studied actual turtles and their gaits all came together to make this happen,” Luis Ramirez GRD ’27, one of the study’s authors, said.
The researchers used a “cost-of-transport” metric — the ratio of power input to the productive power output — to evaluate the efficiency of the robot’s locomotion and compare it to a variety of animals and robots. Using this metric, researchers have found that ART can outperform even exclusively terrestrial or exclusively aquatic robots.
Most notable, however, is the design philosophy behind the robot’s first-of-its-kind morphing ability. The researchers’ pioneering use of adaptive morphogenesis lays the groundwork for the next generation of mobile robots, better equipped to face complex natural environments and their interfaces in the real world.
The study was featured on the cover of Nature on Oct. 12, 2022.